Strategies to mitigate gelatin cross-linking in oral solid drug delivery
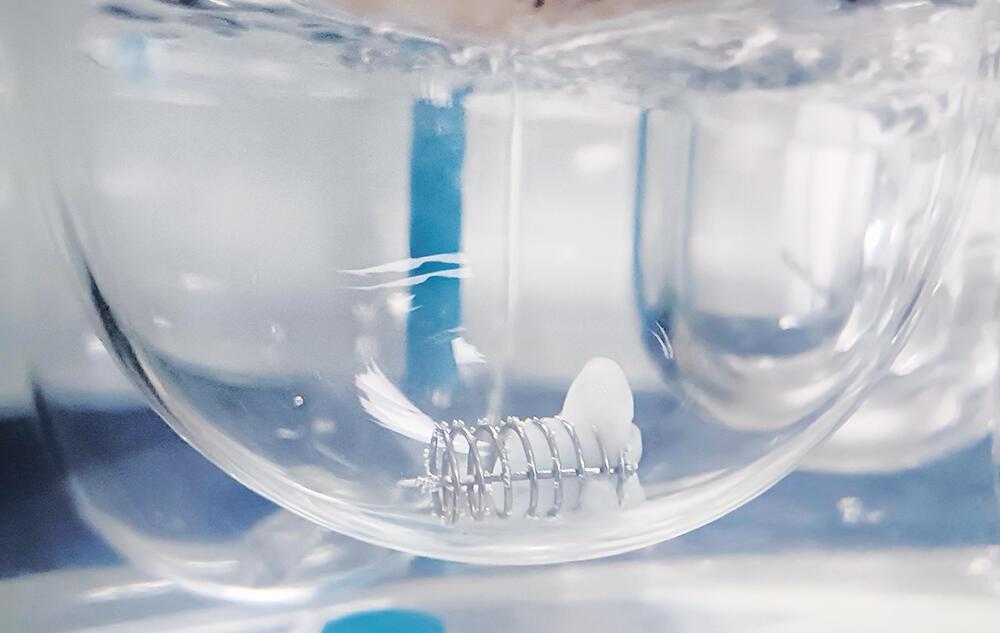
The oral solid drug delivery system is a prominent mode of drug administration. It is recognised for being non-invasive, patient compliant and convenient; steadfastly catering to the multifaceted demands of the market through its pioneering methodologies.
Despite the emergence of cutting-edge techniques such as biologics, molecular delivery and parenteral administration, the prevalence of solid delivery systems continues to undergo ongoing innovation. From traditional pills and capsules to novel variations, such as gummies, soft chews, granules, lozenges and pastilles, the oral delivery mechanism has revolutionised pharmaceutical formulations, capturing the attention of both healthcare professionals and consumers. Its adaptability, accessibility and effectiveness have propelled it to the forefront, surpassing even the established parenteral and intravenous routes.
Irrespective of the product type, the fundamental goal of processing oral solid dosage (OSD) forms remains unwavering: to create formulations that guarantee consistent dosing, reliable distribution of ingredients, and uniform dissolution and bioavailability. However, achieving this objective is contingent upon the dosage forms, actives and excipients employed.
Gelatin often serves as a crucial excipient in OSD formulations. Whether encapsulating active ingredients within hard or soft shells or serving as a coating agent for tablets or a gelling agent in any other formulations, gelatin emerges as a fundamental ingredient across various oral delivery formats. Its versatility finds applications in a wide range of dosage formats.
However, despite its extensive use, OSD manufacturers grapple with a persistent challenge: gelatin cross-linking within formulations, initially observed in 1974 for hard capsules containing chloramphenicol. This phenomenon poses a threat to the dissolution of pharmaceutical products, underscoring the need for strategies to mitigate such barriers. As we navigate the complexities of drug formulations, addressing issues such as gelatin cross-linking becomes paramount in advancing the efficacy and reliability of oral drug delivery systems.
CROSS-LINKING IN THE GELATIN
Following the ingestion of gelatin capsules or gelatin-coated tablets, the primary objective of the formulation lies in releasing the inner content into the biological media. However, it is often observed that gelatin-based formulations tend to experience dissolution failures upon ageing, which is primarily attributed to cross-linking in stressed gelatin-containing products. It causes the formation of a swollen, thin, tough, rubbery, water-insoluble membrane, known as a pellicle
(Figure 1).
This may act as a barrier to the drug release from the formulation, resulting in the slower release of the filled formulation, which consequently leads to a decrease in dissolution values or no release at all in in vitro studies. The degree of cross-linking can vary within and between capsules, making it difficult to predict the extent of the issue. Once formed, these pellicles are irreversible, rendering the gelatin insoluble. As a consequence, dissolution results will have higher variability when gelatin capsule/coated tablets are cross-linked. This phenomenon – during the early stages of drug insertion into the market – often results in failure to meet regulatory guidelines for in vitro studies, prompting regulatory authorities to recall the product.
MECHANISM OF GELATIN CROSS-LINKING
Gelatin, derived from collagen via hydrolysis, is a soluble protein mixture primarily composed of amino acids (Figure 2). These amino acids, linked by amide bonds, form a linear polymer with molecular weights ranging from 15,000 to 250,000. Cross-linking in gelatin can arise from chemical interactions between its peptide chains or individual amino acids. These interactions can be triggered by small amounts of aldehydes, which are present in excipients or APIs. They may also occur from the breakdown of formulation components or packaging materials – or exposure to harsh conditions such as high temperatures and humidity.
Figure 2: Gelatin structure.
There are two main types of cross-linking reactions observed in gelatin: internal and external. Internal cross-linking takes place when gelatin capsules are exposed to high temperatures and humidity, primarily affecting their inner surfaces. In contrast, external cross-linking occurs when gelatin-based formulations, such as hard or soft capsules and gelatin-coated tablets, interact with substances such as aldehydes, peroxides or sulfonic acids.
These substances cause chemical changes in the gelatin molecules, transforming them from a random coil configuration to a collagen triple helix structure.
The mechanism of gelatin cross-linking involves three distinct pathways, each driven by specific molecular interactions and catalytic factors.
- Intrastrand and Interstrand Cross-Linking: Gelatin’s reactivity stems from interactions among amino acids within the same gelatin molecule (intrastrand) or between neighbouring molecules (interstrand). Key amino acids involved in this process (most notably lysine) exhibit trifunctional properties. Lysine residues in close proximity undergo oxidative deamination, yielding terminal aldehyde groups. These aldehyde groups can then react with neighbouring ε-amino groups of lysine, forming imine intermediates. Subsequent aldol condensation reactions lead to the formation of cross-linked products containing pyridinium rings. This cascade of reactions is catalysed by external factors such as heat and relative humidity or the presence of a chemical agent.
- Reaction with Carbonyl Groups: Another mechanism involves the interaction between lysyl ε-amino groups and aldehydic impurities present as contaminants. This reaction generates hydroxymethylamino derivatives, which undergo dehydration to form imines. These imines then engage in further reactions with hydroxymethyl lysine residues, forming dimethyl ether bridges. Ultimately, rearrangement reactions result in methylene linkages between lysyl ε-amino groups, facilitating cross-link formation. Similar reactions can occur with aldose sugars commonly found in pharmaceutical formulations, wherein imine intermediates react with free amino groups to yield ketose sugars. Subsequent reactions between imines and sugars lead to cross-linking via carbonyl functionalities.
- Formation of Aminals: Additionally, gelatin cross-linking can occur through the formation of aminals, which are amine derivatives of acetals. This process is influenced by the pH of the environment. Aminals formed between gelatin chains contribute to cross-linking, further enhancing the structural integrity of the gelatin network.
FACTORS CONTRIBUTING TO GELATIN CROSS-LINKING
Cross-linking in gelatin formulations can stem from various environmental and chemical factors, significantly impacting their stability and performance (Table 1).
Drug | Dosage | USP | Speed | Medium | Volume | Recommended Sampling Times (minutes) | Updated |
Lopinavir/Ritonavir | Capsulte (Soft-Gelatin) | II (Paddle) | 50 | Tier 1: | 900 | 10,15, 30 | 06/18/2007 |
Celecoxib | Capsule | II (Paddle) | 50, 100 | Tier I Medium: | Tier I: 1,000 mL | 10, 20, 30, | 07/01/2010 |
Table 1: Representative two-tier dissolution method.
Environmental Factors
Elevated humidity, temperatures and intense light exposure can trigger cross-linking reactions within gelatin-based formulations, resulting in prolonged in vitro dissolution times. The presence of high humidity facilitates direct catalysis of imine formation, a crucial step in cross-linking reactions.
Additionally, certain excipients may produce byproducts in moist environments, further promoting cross-linking. For example, corn starch may contain hexamethyl tetramine, which decomposes in humidity, generating ammonia and formaldehyde, thus facilitating cross-linking in gelatin. Moreover, high humidity conditions can induce arginine-arginine cross-linking in gelatin. Elevated temperatures accelerate the rate of cross-linking reactions, hastening the formation of insoluble gelatin networks.
Light or ultraviolet (UV)-visible radiation exposure can influence dissolution properties. Intense light exposure correlates with reduced in vitro dissolution rates, adding complexity to the matter of gelatin cross-linking in pharmaceutical products.
Chemical Factors
Several chemical factors can exacerbate gelatin cross-linking in pharmaceutical formulations (Table 2).
Humidity |
Heat |
Light |
Excipients or APIs with |
Aldehydes (furfural, acrolein, formaldehyde, glyceryl aldehyde) |
Imines |
Sugars (glucose and aldose sugar) |
Oxidising agent |
PEGs (containing peroxide/aldehydes) |
Metal ions (colorants/dyes) |
Sulfated polysaccharides |
Table 2: Causative agents for cross-linking.
One prominent contributor is formaldehyde, which can be released from various sources commonly found in formulation excipients. These sources include plasticisers, preservatives and polyethylenated compounds, such as polyethylene glycol (PEG). Interestingly, ethers of PEG, aliphatic alcohols or phenols, polyethylenated glycerides and non-ionic surfactants can all contribute to formaldehyde release, thus promoting the cross-linking of gelatin. PEGs, frequently employed as solvents in pharmaceutical formulations, can release low-molecular-weight aldehydes when exposed to aerobic conditions, thereby further enhancing gelatin cross-linking. Additionally, aldehydes such as furfural, commonly found in bottles containing rayon coils, have been demonstrated to react with gelatin, resulting in the formation of insoluble cross-linked products.
To confirm whether gelatin cross-linking has occurred, instrumental techniques such as various spectroscopies have been applied, including nuclear magnetic resonance (NMR), UV spectroscopy using 2, 4, 6-trinitrobenzene sulfonic acid (TNBS) assay, fluorescence spectrophotometry (FS), near infrared (NIR) and Fourier transform-infrared (FT-IR). However, it is important to note that these spectroscopic techniques may have limitations for identifying cross-linking in gelatin capsule shells when the formulations experience dissolution slowdown due to stress tests in stability studies or a long-time storage of marketed formulations.
STRATEGIES TO MITIGATE THE CHALLENGE POSED BY CROSS-LINKING IN GELATIN-BASED FORMULATIONS
Overcoming the challenge of cross-linking in a dissolution study is crucial for innovators facing hurdles in the in vitro evaluation of gelatin formulations. Fortunately, there are several ways to mitigate the issue of cross-linking within the formulation. One such approach involves leveraging different grades of gelatin, each exhibiting distinct behaviours regarding cross-linking. Notably, type B gelatin demonstrates reduced cross-linking compared with type A gelatin.
Another effective approach involves inhibiting the formation of aldehydes, which are crucial catalysts for cross-linking in capsules. Compounds such as lysine, phenylalanine, glutamine, p-aminobenzoic acid and glycine serve as potent scavengers for carbonyl compounds, obstructing aldehyde interaction with the gelatin shell and thereby significantly reducing cross-linking. Using glycine to scavenge aldehydes in the formulation effectively neutralises reactive aldehydic functional groups, preventing further cross-linking. Furthermore, combining glycine with citric acid proves highly effective, offering dual benefits by scavenging aldehydes and facilitating pH manipulation within the formulation.
Other inhibitors, such as semicarbazide, piperidine and hydrochloride, can also modify capsule properties, effectively inhibiting cross-linking. An alternative approach involves using aldehyde-free excipients, presenting a promising avenue for tackling the dissolution challenge. Importantly, safeguarding formulations against moisture, temperature fluctuations and light exposure will also play a pivotal role in maintaining stability and preventing undesirable cross-linking reactions.
A collaborative effort between the US FDA’s CDER, the United States Pharmacopeia (USP) and academia in the early 1990s yielded valuable insights into gelatin capsule non-compliance during dissolution tests and its impact on bioavailability.1 Notably, while cross-linking primarily occurs in vitro, the behaviour shifts markedly during in vivo testing. Cross-linked capsules face minimal hurdles in vivo, suggesting a divergence between laboratory and real-world outcomes. This is due to the presence of the enzymes in the gastrointestinal (GI) environment acting as biological scissors, effectively breaking down the cross-linked bonds in capsule shells.
This research led to the development of two-tier dissolution testing, which incorporates enzymes into the dissolution medium in some cases to account for potential in vivo enzyme activity, highlighting the importance of considering both in vitro and in vivo data when evaluating drug delivery systems. The USP two-tier dissolution method offers a standardised approach for handling formulations that fail initial dissolution assessments. This methodology aligns well with bioequivalence studies, streamlining processes and reducing associated time and costs.
The USP General Chapters Dissolution <711>and Disintegration and Dissolution of Dietary Supplements <2040> offer provisions for incorporating enzymes into dissolution media when dosage forms fail to meet dissolution acceptance criteria due to gelatin cross-linking. More than 25 pharmaceutical products have used two-tier dissolution methods, as per the FDA’s dissolution database and USP standards (Table 1). In particular, GI enzymes such as pepsin and pancreatin have proven effective in breaking down cross-linked gelatin within the stomach, operating within specific pH ranges to optimise protease activity. By integrating these enzymes into dissolution studies, the observed slow drug release in vitro can be addressed effectively. This strategic inclusion ensures that dissolution assessments accurately reflect the intended performance of the formulation, aligning with regulatory standards and facilitating robust evaluation of product efficacy.
The implementation of two-tier dissolution testing – primarily introduced in the First Supplement of USP 24 – involves the strategic addition of enzymes to dissolution media. Pepsin, added to acidic mediums or water, aims for an activity level of 750,000 units per litre or less. However, it demonstrates optimal proteolytic activity up to pH 4 and diminishes above pH 5.5, rendering it unsuitable for two-tier dissolution testing when the medium exceeds pH 4.
On the other hand, pancreatin USP, included in mediums at or above pH 6.8, targets a protease activity level of not exceeding 1,750 units per litre. Pancreatin, renowned for its wide substrate specificity, boasts enzymes such as trypsin, α-chymotrypsin, carboxypeptidase, lipase and amylase, enabling it to hydrolyse proteins, fats and polysaccharides effectively. With a favourable proteolytic activity range around pH 6–8, pancreatin presents a versatile option for addressing gelatin cross-linking during dissolution testing.
While pepsin and pancreatin offer valuable solutions for in vitro dissolution challenges, it is important to acknowledge their limitations in mimicking in vivo conditions fully. Factors such as the presence of bile salts and gastric motility, crucial in vivo mechanisms, are challenging to replicate accurately in routine dissolution testing. At this point, enzymes such as papain and bromelain, derived from papaya and pineapple, respectively, could be considered. These enzymes, not naturally found in the human body, are primarily employed to digest cross-linked gelatin during dissolution tests. Papain, with an optimal pH range of 4–7, and bromelain, optimal at pH 4.5–7.5, both exhibit potent protease activity and can be evaluated using the case in digestive power test, as delineated in the USP monographs.
While these remedies offer promise in resolving the cross-linking challenge, careful consideration must be given to the cautionary note regarding the use of other additive media components when designing dissolution media. For example, the inclusion of surfactants such as sodium lauryl sulfate for solubility enhancement can compromise the activity of enzymes, introducing complexities in the two-tier dissolution study (Table 1). In such cases, a prudent approach involves pre-treating cross-linked gelatin capsules with enzyme-containing media devoid of surfactants. This ensures the maintenance of enzymatic activity, thus preserving the accuracy of dissolution testing. By navigating these intricacies thoughtfully, researchers could optimise dissolution protocols to emulate physiological conditions, thus enhancing the reliability of their findings more closely.
SUMMARY
While cross-linking in gelatin-based pharmaceuticals may pose challenges to dissolution during in vitro studies, it is important to note that in vivo dissolution is often unaffected. This is due to the enzymatic activity within the GI tract which digests cross-linking efficiently, aiding dissolution. Nonetheless, despite this natural process, several precautions should be taken to ensure regulatory acceptance of the product.
Various strategies exist to prevent cross-linking during the formulation stage. These include tailoring gelatin formulations to specific grades, incorporating cross-linking inhibitors, integrating stability enhancers or exploring alternative excipients less prone to cross-linking. These approaches effectively mitigate cross-linking issues.
However, if cross-linking does occur during formulation, strategic use of the USP two-tier dissolution method would be crucial, especially in capsule formulations. Optimising dissolution studies in this manner can enhance pharmaceutical evaluations, supporting the reliability and efficacy of drug development processes. Through these proactive measures, researchers can confidently and precisely navigate the complexities of cross-linking in gelatin-based pharmaceuticals.
REFERENCE
- Aikman M et al, “Collaborative development of two-tier dissolution testing for gelatin capsules and gelatin-coated tablets using enzyme-containing media”. Pharmacopeial Forum, Vol 24(5), Sep-Oct 1998.